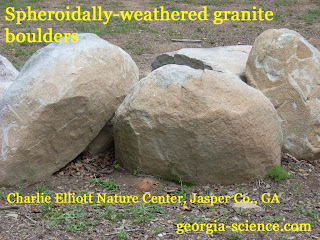
One possibility is that the boulders were rounded in a river channel subject to "big ass" floods, necessary to move and abraid the boulders against each other.
But more often, the boulders are rounded in situ (in place) by a process called spheroidal weathering. On the Georgia Piedmont, most often we see spheroidal weathering in granites and granitic gneisses.
A couple of other places where I have seen spheroidal weathering of granites is Texas Canyon, AZ and Sequoia National Park. [While traveling with my college roommate Dave in 1974, we were in our campsite in Sequoia, stretched out on the hood of the car, leaning against the windshield watching the stars. In the dim light of nearby lanterns, we could see that we were surrounded by rounded granite boulders. After a while, I became aware that there seemed to be an "extra boulder". Turning on the flashlight, that "extra boulder" stood up (it was a bear). At that point, we decided the car was a better place to sleep that night, rather than the tent.]
An additional place where I have seen spheroidal weathering, of volcanic ash-flow tuffs, is in the City of Rocks State Park in New Mexico. [I may place a photo in a later post of this particular place.] With the softer ash-flow tuffs, wind erosion may have also played a role in rounding the boulders, in that particular locale.
What is destined to become rounded boulders starts off as fractured bedrock, broken by what we call brittle deformation. At depth, with the greater confining pressure and heat, rocks become plastic (softened) and undergo folding, stretching, and other forms of ductile deformation. At shallower depths, without the confining pressure and the flexibility provided by the higher temperatures, the rocks become fractured and jointed during earthquakes and other heavings of the earth, leaving angular corners.
As weathering and erosion proceed over the countless millenia, the downward percolating water and natural acids attack the minerals on the outer margins of the rock, by way of the joints and fractures - in the shallow subsurface. The chemical breakdown of minerals by chemical reactions is called chemical weathering.
There are several chemical weathering processes, the one that attacks the feldspars in the granites is primarily hydrolysis.
In areas prone to occasional freezing, the expansion of ice in the fractures (and microfractures) provide even more surface area. This is one process in what we call physical weathering.
Over time, the combined processes of physical and chemical weathering attack the corners of the rock, rounding them off. As erosion carries away overlying soil and degraded rock, the boulders become exposed, wherein the processes of chemical and physical weathering continue, yielding the rounded boulders as seen in the photo.
[The scars seen on the photos are likely from the bulldozers used to "gather" the boulders during construction at the nearby Nature Center parking lot.]
[Visit this post for links to previous posts in this series.]