...in Galveston, Texas. No other descriptions are needed. That is why as Katrina was barreling through the Gulf of Mexico, in 2005, Galveston had a fleet of school buses gassed and ready to go, just in case, while New Orleans' fleet of school buses was awaiting being idle and then flooded. Galveston will probably always be ready, as much as is possible, anytime a hurricane moves westward through the Gulf of Mexico.
Saturday was the anniversary, though I didn't find this Galveston Storm website, until tonight, by way of this Blue Crab Boulevard post.
To provide the context that others miss, we need to remember the death toll of this storm, as recounted on Blue Crab Boulevard:
..."at least 6,000 in Galveston proper and possibly as many as 12,000 in the immediate region."
This was out of a Galveston population of approximately 37,000. Approximately 1 out of every 6 persons died. In contrast, the estimated death toll for the 1906 San Francisco earthquake was 3,000. Adding the death toll from the 1889 Johnstown, PA flood still does not equal the Galveston death toll.
The hurricane changed the course of Texas history. Prior to the hurricane, Galveston was a major economic powerhouse. After the hurricane, economic activity shifted inland to Houston, which was protected from the direct storm surge.
The story of the storm was recounted in the book "Isaac's Storm", named after the U.S. Weather Bureau meteorologist on duty in Galveston, Isaac Cline. The paperback edition of this book, by Erik Larson, was published by Random House, ISBN 0-375-70827-8.
From the Random House website, here is a portion of the narrative on the book:
"'An absurd delusion,' is how Isaac Cline, a dedicated and highly trained first-generation employee of the new U.S. Weather Bureau, characterized the fear that any hurricane posed a serious danger to the burgeoning city of Galveston, Texas. Based partly on Cline's expert opinion, Galveston dismissed a proposal to erect a seawall, claiming it a needless, wasteful expense."...
..."At the turn of the century, Galveston was booming. It was the nation's biggest cotton port, its third-busiest port overall, and the second-most-heavily-traversed entry for immigrants arriving from Europe, nicknamed the "Western Ellis Island." The city had more millionaires, street for street, than any other in America. The nation, too, was bursting at its borders with optimism and confidence."...
Not to minimize the death toll from Hurricane Katrina, but if you hear the characterization of Katrina as "the worst", please endeavor to politely remind the speaker of the 1900 Galveston hurricane, as the human death toll is a measure that should never be forgotten in favor of monetary damages. But to some, what is highlighted depends on the political benefits.
Friday, January 9, 2015
What a Geologist Sees - Part 7
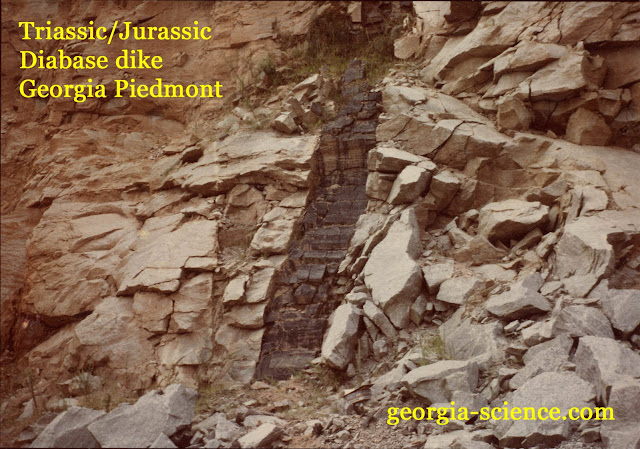
To many geologists, rock quarries are playgrounds, especially if there is more than one type of rock present. Unfortunately, because of today's litigious society, it is difficult to gain access to quarries and to do it without the constraints of a tour group is even more difficult.
My Dad and I initially visited this local "road gravel" quarry about 1975, when you could just drive in there on a Sunday and as long as you stayed away from the machinery and the vertical quarry walls, you were OK.
By the time I went back to do some field work for my "undergrad thesis", during the Summer of 1976, I had to beg for a release form and then I was only allowed about 50 minutes, covering two different visits. Nowadays, the quarry is a very popular place for tours (every Thursday) and because of the popularity with school groups, you have to make reservations about 6 months in advance and for some reason, they don't allow photographs. Hmm. [This photo was taken in 1976.]
The light-colored rock is a metamorphosed granite, called a "gneiss" and it consists of quartz, two different feldspars, and two different micas, along with a host of minor accessory and trace minerals. When rocks are metamorphosed over a large area, this is called "regional metamorphism".
The black-colored rock is a diabase dike. Diabase is a dark-colored, fine-grained igneous rock, similar to basalts that one sees in lava flows in Hawaii, Iceland, New Mexico, Idaho, and elsewhere. It is largely composed of calcium plagioclase feldspar, pyroxene, and olivine.
An igneous dike is a tabular (flat) body of rock that intrudes and cuts-across pre-existing rock and local structures. A tabular body of igneous rock that is parallel to local structures is a sill.
The relationship between the two rock bodies is termed a "cross-cutting relationship", wherein the dike cuts-across the pre-existing rock, thus the dike is the younger rock, even if we do not know the absolute (radiometric) ages of either rock body. In the 1700's, James Hutton recognized this concept.
When this dike (about 3 feet wide) was intruded into the gneiss "country rock" (presumably related to the fracturing of the crust during the rifting of Pangea), the heat of the intrusion triggered some minor changes to the gneiss through "contact metamorphism". This was a "dry intrusion", i.e., it didn't contain much pressurized water, so the "zone of contact metamorphism" in the gneiss - adjacent to the dike - is only about 5 - 6 inches wide. Pressurized water helps ions move around, triggering more mineral changes.
So, with the small width of the intrusion and the paucity of pressurized water, the changes to the gneiss were rather minor. These diabase dikes are common in the Piedmont of Georgia, South Carolina and North Carolina. They can range in width from 3 inches wide in this quarry to more than 1,000 feet in South Carolina. Virtually all of them are oriented NW-SE, cutting across the rest of the regional geologic structures. They are all presumed to have been intruded during the Triassic and Jurassic Periods of the Mesozoic Era (the age of the dinosaurs).
My Dad and I initially visited this local "road gravel" quarry about 1975, when you could just drive in there on a Sunday and as long as you stayed away from the machinery and the vertical quarry walls, you were OK.
By the time I went back to do some field work for my "undergrad thesis", during the Summer of 1976, I had to beg for a release form and then I was only allowed about 50 minutes, covering two different visits. Nowadays, the quarry is a very popular place for tours (every Thursday) and because of the popularity with school groups, you have to make reservations about 6 months in advance and for some reason, they don't allow photographs. Hmm. [This photo was taken in 1976.]
The light-colored rock is a metamorphosed granite, called a "gneiss" and it consists of quartz, two different feldspars, and two different micas, along with a host of minor accessory and trace minerals. When rocks are metamorphosed over a large area, this is called "regional metamorphism".
The black-colored rock is a diabase dike. Diabase is a dark-colored, fine-grained igneous rock, similar to basalts that one sees in lava flows in Hawaii, Iceland, New Mexico, Idaho, and elsewhere. It is largely composed of calcium plagioclase feldspar, pyroxene, and olivine.
An igneous dike is a tabular (flat) body of rock that intrudes and cuts-across pre-existing rock and local structures. A tabular body of igneous rock that is parallel to local structures is a sill.
The relationship between the two rock bodies is termed a "cross-cutting relationship", wherein the dike cuts-across the pre-existing rock, thus the dike is the younger rock, even if we do not know the absolute (radiometric) ages of either rock body. In the 1700's, James Hutton recognized this concept.
When this dike (about 3 feet wide) was intruded into the gneiss "country rock" (presumably related to the fracturing of the crust during the rifting of Pangea), the heat of the intrusion triggered some minor changes to the gneiss through "contact metamorphism". This was a "dry intrusion", i.e., it didn't contain much pressurized water, so the "zone of contact metamorphism" in the gneiss - adjacent to the dike - is only about 5 - 6 inches wide. Pressurized water helps ions move around, triggering more mineral changes.
So, with the small width of the intrusion and the paucity of pressurized water, the changes to the gneiss were rather minor. These diabase dikes are common in the Piedmont of Georgia, South Carolina and North Carolina. They can range in width from 3 inches wide in this quarry to more than 1,000 feet in South Carolina. Virtually all of them are oriented NW-SE, cutting across the rest of the regional geologic structures. They are all presumed to have been intruded during the Triassic and Jurassic Periods of the Mesozoic Era (the age of the dinosaurs).
What a Geologist Sees - Part 8
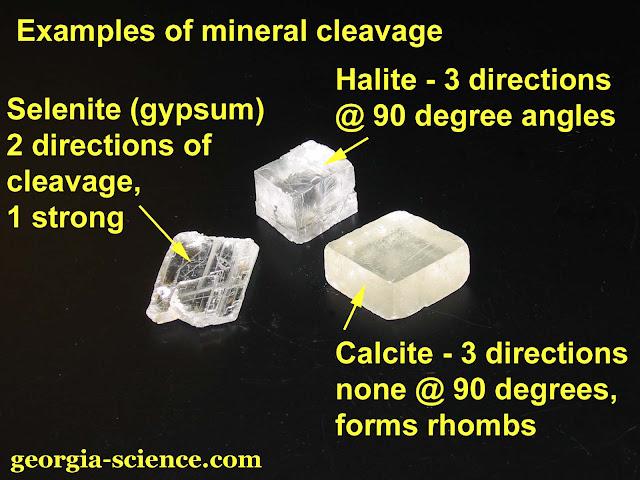
Despite all of the fancy lab equipment these days, it is still necessary for a geologist to often make field identifications of minerals and rocks.
As mineral color may be affected by impurities (trace elements), one of the diagnostics that we use for mineral identification is "mineral cleavage".
Cleavage is the characteristic by which when a mineral breaks, it leaves behind a flat surface. The number of cleavage directions and the quality of the cleavage (on any and all of these cleavage directions) is affected by the internal structure of the mineral. Perfect cleavage in a mineral is because of inherent planes of weakness within the crystal structure (lattice). Micas are examples of minerals with one direction of perfect cleavage and cleavage surfaces are parallel to each other, on opposite sides of a mineral specimen.
Various minerals can have 1, 2, 3, 4, even 6 directions of cleavage (as in Sphalerite - the zinc, iron sulfide mined on the Gore family property in Tennessee). In minerals with more than one direction of cleavage, the angles between the cleavage planes are important.
In the examples above, we have three common minerals, all of which may be transparent to translucent and colorless in the absence of impurities. One way of distinguishing between them is to recognize the differences in cleavage (as explained in the photo). Halite (table salt) breaks into almost perfect cubes with 90-degree angles between all three directions of cleavage - which we call "cubic cleavage". Galena, a heavy, silvery lead sulfide also has cubic cleavage.
Calcite has three directions of cleavage, but none of them are at 90 degree angles to the others. This we call "rhombic cleavage".
Selenite (gypsum) occurs in several forms, in this clear crystalline form, it's one strong direction of cleavage causes it to break into thin, flat sheets, while a secondary, weaker direction of cleavage affects the shape of the specimen margins. [Hint: Selenite is one of only two common minerals that are softer than your fingernail, i.e., you can scratch it.] Differing degrees of Hardness (resistance to scratching) can be used also as a diagnostic tool. [That may be covered in a future post.]
If a broken surface is not smooth enough to classify as a cleavage surface, then it is termed as "fracture". Quartz is an important mineral that does not have cleavage (some suggest it has a very weak cleavage, but for the sake of simplicity, we will stick with the concept of no cleavage for quartz). Pyrite, olivine (peridot) and garnets are examples of other minerals with no cleavage. Some minerals, especially in the "quartz family" exhibit a distinctive type of fracture - "conchoidinal fracture", the curving type of fracture that you see in glass. The fracture link explains some of the other types of mineral fracture.
Geology students commonly study individual minerals first, in hand-size samples (if possible) to become familiar with the cleavage and other characteristics of the minerals, before we start studying minerals as smaller components of rocks. Usually we study igneous rocks after minerals, as igneous rocks are the original source of most minerals.
Cleavage can have a larger impact than some imagine. When flat, platy minerals such as micas are present in certain rocks, especially metamorphic rocks, if they are aligned with each other, they can form zones of weakness, which can affect the way rocks break. Knowing the cleavage characteristics in a diamond is important as it affects how a larger diamond can be split into to numerous smaller diamonds for faceting purposes.
As mineral color may be affected by impurities (trace elements), one of the diagnostics that we use for mineral identification is "mineral cleavage".
Cleavage is the characteristic by which when a mineral breaks, it leaves behind a flat surface. The number of cleavage directions and the quality of the cleavage (on any and all of these cleavage directions) is affected by the internal structure of the mineral. Perfect cleavage in a mineral is because of inherent planes of weakness within the crystal structure (lattice). Micas are examples of minerals with one direction of perfect cleavage and cleavage surfaces are parallel to each other, on opposite sides of a mineral specimen.
Various minerals can have 1, 2, 3, 4, even 6 directions of cleavage (as in Sphalerite - the zinc, iron sulfide mined on the Gore family property in Tennessee). In minerals with more than one direction of cleavage, the angles between the cleavage planes are important.
In the examples above, we have three common minerals, all of which may be transparent to translucent and colorless in the absence of impurities. One way of distinguishing between them is to recognize the differences in cleavage (as explained in the photo). Halite (table salt) breaks into almost perfect cubes with 90-degree angles between all three directions of cleavage - which we call "cubic cleavage". Galena, a heavy, silvery lead sulfide also has cubic cleavage.
Calcite has three directions of cleavage, but none of them are at 90 degree angles to the others. This we call "rhombic cleavage".
Selenite (gypsum) occurs in several forms, in this clear crystalline form, it's one strong direction of cleavage causes it to break into thin, flat sheets, while a secondary, weaker direction of cleavage affects the shape of the specimen margins. [Hint: Selenite is one of only two common minerals that are softer than your fingernail, i.e., you can scratch it.] Differing degrees of Hardness (resistance to scratching) can be used also as a diagnostic tool. [That may be covered in a future post.]
If a broken surface is not smooth enough to classify as a cleavage surface, then it is termed as "fracture". Quartz is an important mineral that does not have cleavage (some suggest it has a very weak cleavage, but for the sake of simplicity, we will stick with the concept of no cleavage for quartz). Pyrite, olivine (peridot) and garnets are examples of other minerals with no cleavage. Some minerals, especially in the "quartz family" exhibit a distinctive type of fracture - "conchoidinal fracture", the curving type of fracture that you see in glass. The fracture link explains some of the other types of mineral fracture.
Geology students commonly study individual minerals first, in hand-size samples (if possible) to become familiar with the cleavage and other characteristics of the minerals, before we start studying minerals as smaller components of rocks. Usually we study igneous rocks after minerals, as igneous rocks are the original source of most minerals.
Cleavage can have a larger impact than some imagine. When flat, platy minerals such as micas are present in certain rocks, especially metamorphic rocks, if they are aligned with each other, they can form zones of weakness, which can affect the way rocks break. Knowing the cleavage characteristics in a diamond is important as it affects how a larger diamond can be split into to numerous smaller diamonds for faceting purposes.
What a Geologist Sees - Part 9
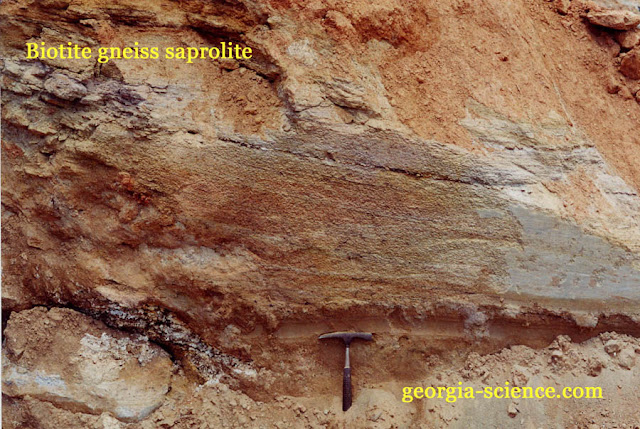
Figure 1.
The photo at top is of an embankment of saprolite, which is literally (and colloquially called) "rotten rock". This embankment was exposed during a short period of local construction. Saprolite is the term used to describe exposures that display some original rock structures, but because of intense chemical weathering, the internal structures of most of the minerals have been broken down to the point where the "rock" can be crumbled in your hand - thus the term "rotten rock". The processes that yield saprolite are what produce the inorganic components of soils.
If the exposure has been reduced to a structureless clay, e.g., the famous Georgia red clay, we call this "residuum".
The lower photo is of the original "country rock", a fresh, hard biotite gneiss, a metamorphic rock that is similar to granite. This particular gneiss (silent "g", rhymes with "rice"), probably part of the widespread Lithonia Gneiss, is composed of potassium (orthoclase) feldspar, plagioclase feldspar, quartz, biotite mica and probably muscovite mica, along with some accessory and trace minerals.
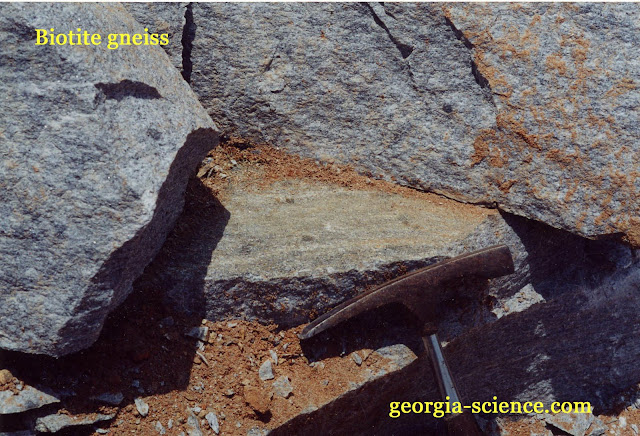
Figure 2.
The progression from gneiss to saprolite takes place through chemical reactions involving water and natural acids over long periods of time. In the process of hydrolysis, hydrogen cations replace the metallic ions (K, Na, Ca) that occur in the mineral structures of feldspars, micas, and other silicate minerals. The removed metallic ions then become part of the dissolved minerals in groundwater (and surface waters) and are flushed out of the system. Any iron-bearing minerals, including silicates, are altered by oxidation, in addition to hydrolysis. There are other chemical weathering processes, but these two are probably most prevalent.
At or near the surface, quartz is generally fairly stable, so probably most of the original quartz remains, while the feldspars and micas have broken down into various clays.
In addition to the chemical weathering, whereby the actual chemistry of the minerals change, there is also physical weathering, where the rock is broken into smaller pieces by natural processes, yielding more surface area upon which the chemical processes can occur, i.e., both types can occur simultaneously and tend to enhance one another.
It is a little unusual, in this area, to see the fresh parent rock and the resulting saprolite in the small area of a stand-alone drug store.
On a side issue, if there is enough quartz present (as opposed to clays) and there is enough porosity and permeability (hydraulic conductivity), saprolites can sustain small, residential drinking water wells, in this area.
What a Geologist Sees - Part 10 (During drought conditions in 2007)
At Laurel Park in Hall County, GA, u
p the hill from the old raceway grandstands - in a small cliff that normally marks the edge of the peninsula at Laurel Park - is this outcrop of old river gravels overlying saprolite, similar to what I wrote about in "What a Geologist Sees - Part 4". The previous examples of old river gravels over saprolite are on the west side of Duluth, also associated with the Chattahoochee River.
To refresh your memory, saprolite is the heavily-weathered "rotten rock" that has had its structural integrity destroyed by mineral dissolution (actually conversion to clays by hydrolysis). The term is usually used to describe former igneous and metamorphic rocks and traces of the original textures and structures must be discernible, otherwise we call it "residuum" - in this area, that would be Georgia red clay.
This hillside exposure of the old Chattahoochee River gravels overlying saprolite is an example of a "topographic inversion", i.e., this used to be the bottom of the river, in the lowest part of the valley. Now it is on a low hilltop, perhaps 1/4 to 3/8 of a mile from the river channel (prior to the filling of Lake Lanier in the late 1950s). A rough guess might be that these gravels are probably some 30-40 feet above the now-covered river bed.
On the opposite side of the peninsula, these river gravels have been eroded and the exposed shoreline is covered with the rounded pebbles and cobbles. I am considering doing a little gold panning, from sand trapped in dips in the exposed bedrock along the shoreline, as the Chattahoochee drainage basin includes Dukes Creek (near Helen, GA), which is a gold-producing area. The idea is just to satisfy my curiosity. There are areas in which old river gravels have been mined for gold.
Again, I bring this up as a way to pique someone's curiosity. Most people don't give a thought to the river ever being anywhere but besides its current floodplain. What did the land look like before that 30-40 (maybe more) feet of vertical down-cutting took place, along with the lateral migration of the river? Questions such as these might arouse a kid's interest in science. In walking the drought-exposed shoreline, I am sure that numerous people every day see the gravels overlying the weathered bedrock, but I doubt that it really "sinks in" as to what it really means.
[I am so easily entertained.]
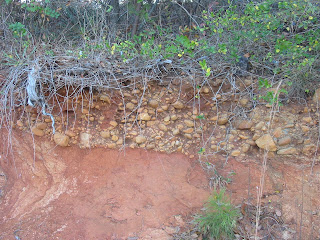
To refresh your memory, saprolite is the heavily-weathered "rotten rock" that has had its structural integrity destroyed by mineral dissolution (actually conversion to clays by hydrolysis). The term is usually used to describe former igneous and metamorphic rocks and traces of the original textures and structures must be discernible, otherwise we call it "residuum" - in this area, that would be Georgia red clay.
This hillside exposure of the old Chattahoochee River gravels overlying saprolite is an example of a "topographic inversion", i.e., this used to be the bottom of the river, in the lowest part of the valley. Now it is on a low hilltop, perhaps 1/4 to 3/8 of a mile from the river channel (prior to the filling of Lake Lanier in the late 1950s). A rough guess might be that these gravels are probably some 30-40 feet above the now-covered river bed.
On the opposite side of the peninsula, these river gravels have been eroded and the exposed shoreline is covered with the rounded pebbles and cobbles. I am considering doing a little gold panning, from sand trapped in dips in the exposed bedrock along the shoreline, as the Chattahoochee drainage basin includes Dukes Creek (near Helen, GA), which is a gold-producing area. The idea is just to satisfy my curiosity. There are areas in which old river gravels have been mined for gold.
Again, I bring this up as a way to pique someone's curiosity. Most people don't give a thought to the river ever being anywhere but besides its current floodplain. What did the land look like before that 30-40 (maybe more) feet of vertical down-cutting took place, along with the lateral migration of the river? Questions such as these might arouse a kid's interest in science. In walking the drought-exposed shoreline, I am sure that numerous people every day see the gravels overlying the weathered bedrock, but I doubt that it really "sinks in" as to what it really means.
[I am so easily entertained.]
What a Geologist Sees - Part 10B (During drought conditions in 2007)
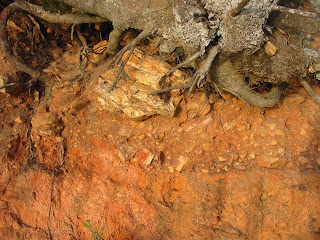
I used to think river gravels were boring stuff (as compared to collecting fossils or neat mineral specimens) until I learned more about old river terraces (floodplain remnants) and exposed gravels on hilltops and hillsides. In these settings, the gravels tell us about how much a long-existing river can migrate laterally over time.
These gravels are on the opposite side of the peninsula from the old raceway grandstands. The peninsula lies within an incised meander of the Chattahoochee River and the old river channel cuts across the hill. Meanders are usually present in areas of low-gradient, usually on coastal plains, closer to the ocean. That it is an incised meander suggests that the meander system was locally established (as just described), then with a rapid drop in base-level, down-cutting preserved the meanders.
Where there are sedimentary rocks in the drainage basin, sometimes you can find petrified (permineralized) wood in river gravels. I used to find small rounded pieces of petrified wood in some of the old Rio Grande gravels west of El Paso.
I hope to return to the park to check out the large chunk of rock pictured just below the tree roots. From the surrounding river pebbles, you can see that this chunk is far larger than the rest, suggesting that "there is more to this story". It would take a great deal more energy to move this size rock, as opposed to the adjacent pebbles. In the exposed gravels along the edges of this peninsula, I don't recall having seen other clasts this large.
There are several possibilities.
[1] A possibility is that this chunk of rock fell into the river from a nearby (now eroded) bluff or rolled down a slope into the river.
[2] Another more interesting possiblity is that this is a chunk of permineralized wood, i.e., a chunk of wood that was buried in the river gravels and mud and was permineralized by silica in the groundwater after burial. I considered that when I shot the photo, but sundown was approaching and the park was about to close, so I didn't remove it from its position to more closely observe the characteristics. A chunk of wood that large would be light enough to be moved along with the gravels, prior to its permineralization.
If it was permineralized wood, "woodn't" that be neat! [Sorry.] As that sort of thing is not that common on the Piedmont, I would take some more photos before dislodging it from the vertical face. Something like that might be worthy of a short scientific paper, perhaps if any internal cell walls are preserved, a paleo-botanist might be able to determine the genus of the wood, based upon the internal structures.
[3] One more possibility is that on the nearby exposed shoreline, amid the weathered, saprolitized metamorphic rocks, there are areas of hard quartzite. This chunk of rock could simply be a chunk of quartzite that was "ripped" from the river floor (you can see the scoured bottom below the gravels) by a flood and "rolled" to its present location. It is easier for fast-moving water to roll an object than it is to carry it.
[There, without your realizing it, I have "walked you through" the concept of "Multiple Working Hypotheses".]
I have proposed three possible ways that this large chunk of rock (maximum dimension is perhaps 1.5 feet) came to be placed within these smaller pebbles in the old river bottom. There could be other possibilities that I have not yet considered. Speaking from my bias as a field scientist, this is why we learn to "brainstorm" with other scientists and when properly trained, we engage our own imaginations. If the chunk of rock is not permineralized wood, then that Hypothesis is discarded and the others subjected to greater scrutiny. That is the way science works. And because of the ravages of time and erosion, there may not be a single "best" answer.
Unlike lawyers and politicians, scientists should understand that our initial opinion may not be the correct one.
Just to Give the Claustrophobes - Including Me - a Little Chill... (Late 2007)
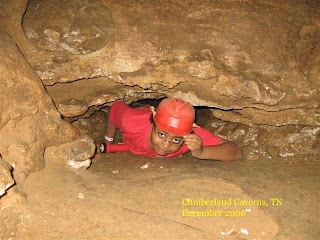
I was bravely holding down a picnic table in the commons area of the caverns at the time. I know my limits. One of the dads that did the crawl admitted afterwards that he started to panic a little, but kept it under control.
On one of these tours, you cain't turn around and go back. It ain't like an outdoor hike where you can turn around and go back after 1/2 mile if you decide you ain't up for it. Or if your knee is getting gimpy.
So to all those that can do this, more power to you. Maybe because Cumberland was drier than Raccoon Mt., there was less slipping and banging of knees. My son said he preferred this dry "wild tour" of Cumberland Caverns to the muddier, more slippery Raccoon Mountain Caverns tour.
I guess I should have taken some crawling photos in Raccoon Mt., but when I am doing that and pushing a duffel bag, I am thinking "I want out", not "Gee, this is a Kodak moment".
What a Geologist Sees - Part 11, A "Bonanza Slab"
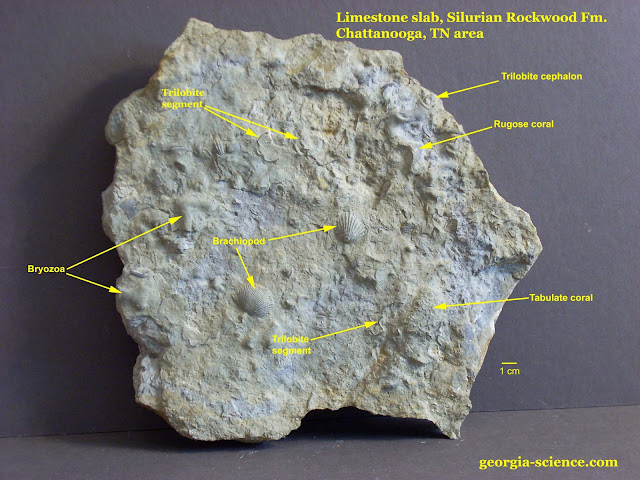
Figure 1.
As a teacher, I would call this a "Bonanza slab", as there are several types of fossils on a single limestone slab. It illustrates the concept of biodiversity (or species diversity) in a Sub-Tropical shallow continental shelf setting or perhaps a shallow carbonate platform setting.
This limestone slab is from the Silurian Rockwood Formation exposed west of Chattanooga, TN, off I-24. Among the more easily seen fossils are brachiopods, rugose corals, tabulate corals, bryozoa, and trilobite fragments. There are fragments of smaller fossils, such as stalked echinoderms.
According to a professor at Univ. of Tennessee - Chattanooga, the Rockwood Formation is an offshore equivalent of the Red Mountain Formation, which consists of red shales, siltstones, and sandstones deposited in tidal flat and delta environments. The Red Mountain Formation was deposited due to the erosion of the Taconic Highlands, which lay to the present-day east and southeast. The Taconic Highlands, uplifted during the Late Ordovician Period represented the first uplift of what would become (much later) the Appalachian Mountains.
What a Geologist Sees - Part 12
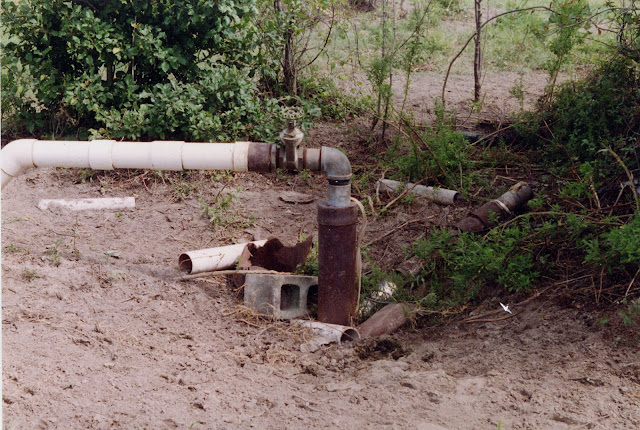
Figure 1.
Just a brief lesson on water well construction and aquifers and other stuff like that.
The upper photo is of a poorly constructed/ poorly maintained residential drinking water well, probably on the order of 120-150 feet deep. It was located on the inner Coastal Plain, a few miles south of Augusta, GA, in Burke County.
Though we (the Georgia Geologic Survey Tritium Project) never subjected this particular well to a detailed examination by geophysical logging, it probably "bottomed out" and was drawing water from one of several formations in the unconfined Upper Three Runs Aquifer.
These could include the Late Eocene Utley Limestone Member of the Clinchfield Formation, Griffins Landing Member of the Dry Branch Formation, or the Irwinton Sand Member of the Dry Branch Formation. Overlying the Dry Branch Formation was the Late Eocene Tobacco Road Sand and that is what makes up the sandy soils seen surrounding the wellhead.
There are two areas of concern regarding the construction of this well. The first involves the apparent lack of a "grout seal". When a drill rig drills a borehole, because of the sloughing of sediments, the borehole is rarely ever a perfect cylinder.
To prevent the sloughing of sediments that would fill the well, usually well casing is installed consisting of Schedule 40 or Schedule 80 (for deeper wells) PVC pipe that is from 4 to 8 inches in diameter. With this well, the rust on the exposed casing suggests a steel or cast iron casing (it has been almost 10 years since I worked this area).
With the installation of the casing, the area between the borehole and the casing is called the "annular space" and it must be properly sealed to prevent surface pollutants from reaching the aquifer. Around the "screen zone", where the water enters the well casing, coarse sand (sand pack or gravel pack) is usually introduced to keep the water flowing from the aquifer into screen zone. Above the "gravel pack" is where the first seal should be applied.
Below the water table, pellets of bentonite clay are introduced by way of a small pipe from the surface. Once wet, the bentonite pellets will swell and seal the annular space, if properly applied. Above the water table and all the way to the surface, a thin slurry of concrete seals the rest of the annular space. At the surface, the well owner is supposed to pour a concrete surface pad to act as the first line of defense against surface pollutants reaching the aquifer.
That is how it should be. A closer look at the surface area (amid the debris) surrounding the wellhead (in the upper photo) suggests that the area slopes inward towards the well casing. This is not good. This suggests that rainwater has been washing the sandy soil into the ungrouted (or poorly grouted) annular space. Along with the sand, whatever else might be on the ground surface (chicken poop, etc.) is susceptible to being washed down the annular space, possibly reaching the aquifer if there is no grouting at all.
If you are ever in the position of buying a piece of property and the wellhead looks like this, at least get the water tested by the county health department or walk away from the deal. Due Diligence applies to things more than land titles and termites.
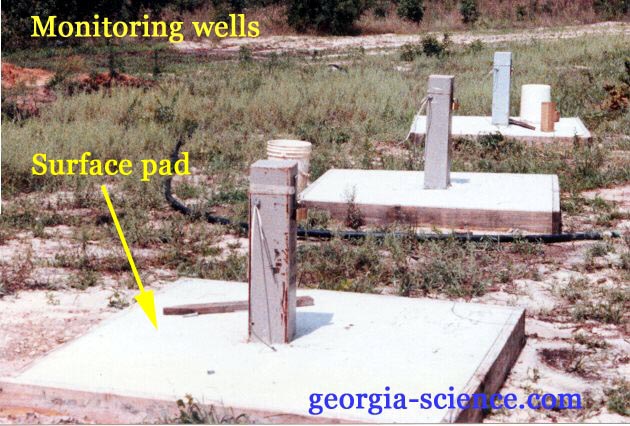
Figure 2.
In the photo above, these three GGS monitoring wells (one mile or so from the above well) exhibit properly installed surface pads to top off the annular space grouting. [There are three wells drilled into three separate aquifers at different depths to test for tritium among other things.
Above-background (but below EPA MCL) levels of tritium were found in the shallow aquifer well (the same one serving the well in the upper photo). One goal of our study was to make sure that similar levels of tritium had not reached the deeper aquifers (which it had not).
If the residential well (drilled to serve a couple of trailers) had at least a surface pad, that would have offered some protection from surface pollutants. Just a reminder, if you are going to purchase a homesite dependent on a water well, you should have it inspected before you commit to a purchase.
What a Geologist Sees - Part 13
One of the many interesting geologic features of the greater El Paso area is Kilbourne's Hole, located approximately 30 miles WNW of El Paso, on the La Mesa Surface (a plateau), in southern Doña Ana County, NM.
This area lies within the Rio Grande Rift and is included in the Potrillo Volcanic Field, which covers approximately 500 square miles. Some of the northernmost Potrillo cinder cones are visible on the southside of I-10, west of Las Cruces, NM.
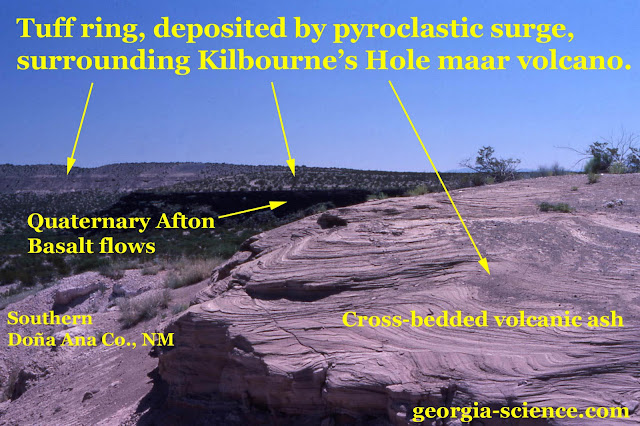
Figure 1.
Kilbourne's Hole is a low-relief, asymmetrical volcanic crater referred to as a "maar" volcano. It measures approximately 1.7 miles by 1 mile (see the first link for an aerial photo). A short distance south lies a smaller maar, Hunt's Hole. The two maar volcanoes lie within the southern end of a small, triangular-shaped graben basin, bounded on the west by the Robledo Fault and on the east by the Fitzgerald Fault (the two faults converge near the Mexican border). To the north-northeast of Kilbourne's Hole lie the Gardner Cones and the Afton Basalts.
The distal ends of some of the Afton flows are visible in the background of the upper photo and covered at least a portion of what is now Kilbourne's Hole, before Kilbourne's Hole was formed. Due north of Kilbourne's Hole lie the Aden Basalts (where I did my Master's Thesis) and Aden Crater, a small shield volcano at the NW "corner" of the Aden Basalts. [Most of the Aden Basalts were erupted from "fissure eruptions" and are termed "flood basalts.]
For years, the origins of Kilbourne's Hole and Hunt's Hole were the subject of much speculation, including their being meteor-impact craters. The formation of a maar in the Philippines in the 1960s provided the needed answers.
Evidence suggests that Kilbourne's Hole formed as the result of repeated, large steam explosions, probably over the course of a few weeks to a few months.
The Aden and Afton Basalts were erupted onto the surface of the Camp Rice Formation, which includes river sediments from the prior meandering of the Rio Grande River during the Pliocene and Pleistocene Epochs of the late Tertiary Period/early Quaternary Period. When buried, river gravels and sand may serve as aquifers and it is believed that as a rising magma body contacted a shallow sand aquifer and the resulting steam explosions produced the crater. [These explosions are termed "phreato-magmatic explosions".]
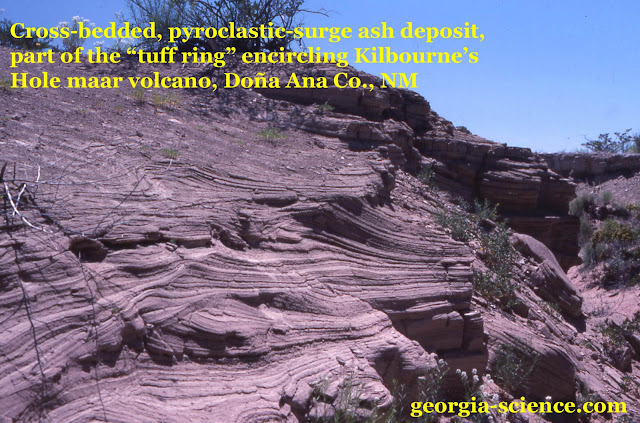
Figure 2.
Normally, basaltic eruptions like those in Hawaii and Iceland, do not produce much ash, unless there are phreatomagmatic explosions. When the explosions take place, the steam explosions pulverize the erupting lava before it can reach the vent, producing ash that is composed of minute particles of volcanic glass, rock fragments, crystals, and dust (each ash eruption will have different percentages of these components).
With some maar eruptions, a pyroclastic "tuff ring" of ash forms around the margins of the maar. The deposition of the tuff ring is probably at least partially controlled by prevailing winds during the explosive events.
The ash itself is deposited as a pyroclastic "base surge" from; 1) The direct lateral components of explosive events; and 2) As lateral deposits around the maar after the collapse of the vertical ash column, i.e., after the explosions, some of the ash remains in the troposphere as "pyrocumulus" clouds and the rest collapses and spreads as it reachs the ground.
The undulating "cross-bedding" of the ash, seen in the photos, is produced by the formation and migration of ash dunes and ripples during and shortly after the eruptive events. The tuff ring is deposited on top of the Afton Basalt flows and exposures of the alluvial Pliocene-Pleistocene Camp Rice Formation. The broad alluvial plain beneath the Aden and Afton Basalts is locally referred to as the "La Mesa Surface" or perhaps the "La Mesa Plateau".
Figure 3.
Also produced by the explosions are ejecta, aka "volcanic bomb1s". In the case of Kilbourne's Hole (Figure 3), the volcanic bombs are sometimes cored by "mantle xenoliths", i.e., pieces of the Earth's mantle (carried in the magma/lava) that were already solidified before they reached the surface.
When broken open, these mantle xenoliths reveal their composition of olivine and enstatite. When the olivine grains are big enough and of the proper color and clarity, they are termed "peridot" and some of the Kilbourne's Hole xenoliths have produced good-quality peridot.
[I wish I had collected more of these volcanic bombs when I lived in the El Paso area and I wish I had taken more slides of Kilbourne's Hole while I was working to the north in the Aden Basalts. I did collect some different types of volcanic bombs from within Aden Crater and from another maar to the west, Riley Maar.]
[I am not sure if there has been a Master's Thesis done on an analysis of the tuff rings and the distribution of the volcanic bombs at Kilbourne's Hole.]
All of the features within the Aden-Afton graben are geologically young, less than 2 million years in age, erupted during the Pleistocene Epoch of the Quaternary Period. These volcanic features and the others of the Potrillo Volcanic Field are related to the crustal thinning of the Rio Grande Rift, a crustal feature which is thought to extend from (approximately) the Big Bend area of Texas/Mexico northward into south-central Colorado (some interpretations may vary).
An Update...
I am reposting "geophotos" (with explanations) from another blog, which is slated for retirement next month. Scroll through as you wish.
What a Geologist Sees - Part 14
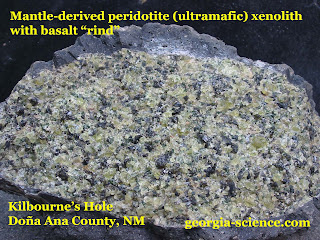
As a follow-up to Part 13, the upper photo is of a broken "volcanic bomb" from Kilbourne's Hole maar volcano, showing the mantle xenolith within. The average mineral grain-size within the xenolith is approximately 2 mm in diameter. The rock peridotite is generally composed of olivine and a pyroxene, in the case of Kilbourne's Hole, I think the pyroxene is enstatite. The composition of the peridotite xenolith defines it as being "ultramafic". The basalt that encloses the xenolith is defined as "mafic". The ultramafic minerals are the first to solidify in a cooling magma, followed by the mafic minerals, which include olivine and pyroxene, as well as some amphiboles and calcium plagioclase feldspars. The slow rate of cooling is responsible for the visible grain sizes in these xenoliths contrasted with the more rapid rate of cooling of the enclosing basalt lava.
In the few places in the world where these types of xenoliths have been erupted, the xenoliths provide a little insight as to the mineral composition of the upper mantle. In both cases shown here, the matrix or host rock is a basalt.
One other mantle xenolith locality in the western United States is San Carlos, AZ, on the Apache reservation east of Phoenix. The photo below is of a sample of the San Carlos xenoliths.
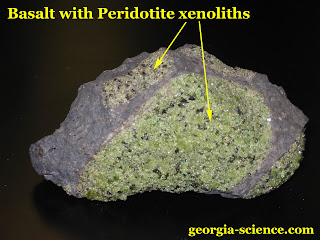
In "What a Geologist Sees - Part 3", I described the concept of "Inclusions", which originated with James Hutton and Charles Lyell. Inclusions, such as these xenoliths, are older than the rock itself, i.e., the solidification of the xenoliths had already taken place when they were included in the rising magma body.
What a Geologist Sees - Part 15
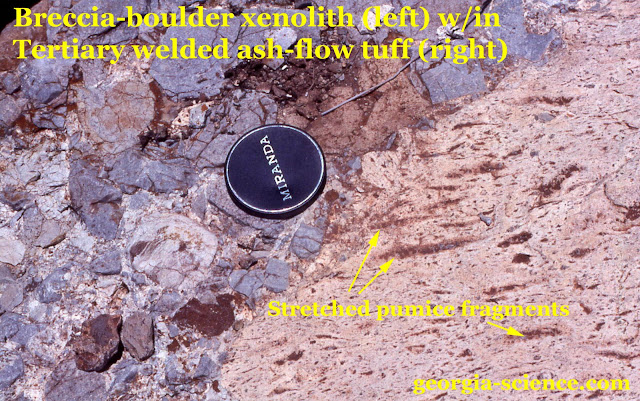
Figure 1.
Inclusions and xenoliths. [OK, ya'll. Next time you play Scrabble, if you wind up with an "x", if you can cobble together "xenolith", that should score a lot of points.]
When there are individual components of an igneous or sedimentary rock that markedly contrast with the matrix, the inquiring mind wants to know - where did that (or those) come from? When the contrasting piece is within an igneous rock, it is a xenolith, as highlighted in other posts in this series. When the contrasting piece occurs within sedimentary rock, it is an inclusion.
With the upper slide, it is in the Eagle Mts. in West Texas. The matrix is on the right, while the xenolith (composed of inclusions) is on the left. The lens cap shows the scale of the inclusions within the breccia boulder that was incorporated into pyroclastic flow. The breccia boulder is older than the pyroclastic matrix and the breccia clasts (pieces) are older than the breccia boulder itself. The pyroclastic ash flows produce the welded ash-flow tuffs when the ash flow movement ends. [That is for a separate post.]
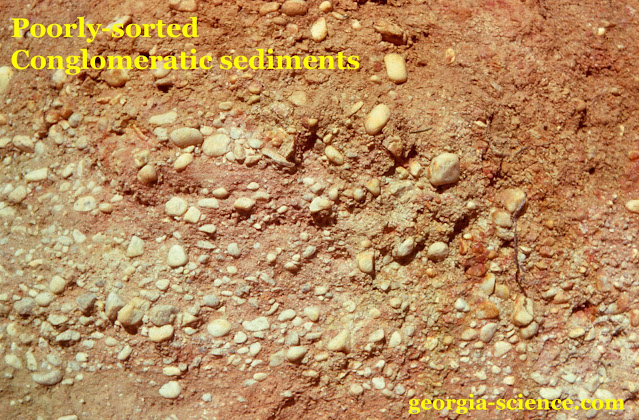
Figure 2.
The lower photo shows conglomeratic sediments. Each pebble came from a rock older than the conglomerate to which it now belongs. In this case, all of the quartzite pebbles in this conglomerate seem to be from a similar source, but sometimes some conglomerates (with different source rocks) can be quite colorful.
[Before going further, a breccia is composed of angular gravel-sized pieces of rock. A conglomerate is composed of rounded gravel-sized pieces of rock.]
Most conglomerates are associated with old river-channel deposits, the conglomerate in the lower photo represents old Chattahoochee River deposits, out of the current river channel.
Because of their angular corners and edges, breccias are generally found near where they are formed. The rock that produced the angular pieces can be broken by a number of different processes including landslides, faulting, meteor impact, and volcanic explosions (the probable source of the breccia in the upper photo, as this photo was taken inside of an old caldera-type volcano, where explosions would be the rule, rather than the exception).
As stated before, both examples illustrate the Concept of Inclusions that originated with James Hutton and Charles Lyell as one method of determining a relative timeline, without knowing the absolute age of any of the events. With the upper photo, there are at least four events recorded; 1) The brecciation (breakage) of the rock fragments within the boulder; 2) The cementation of the angular breccia fragments; 3) The separation of the boulder from its original locality; and 4) Inclusion of the boulder in the pyroclastic ash flow.
As part of another timeline, the stretched, brown pumice fragments existed before the pyroclastic flow, so they are also xenoliths, of a sort.
Subscribe to:
Posts (Atom)